The Shaw Prize was established in 2002. It consists of three annual prizes: Astronomy, Life Science and Medicine, and Mathematical Sciences. It honours individuals who have recently achieved significant breakthrough in academic and scientific research or applications and whose work has resulted in a positive and profound impact on mankind.
The Shaw Prize in Astronomy 2020 is awarded to Roger D Blandford for his foundational contributions to theoretical astrophysics, especially concerning the fundamental understanding of active galactic nuclei, the formation and collimation of relativistic jets, the energy extraction mechanism from black holes, and the acceleration of particles in shocks and their relevant radiation mechanisms.
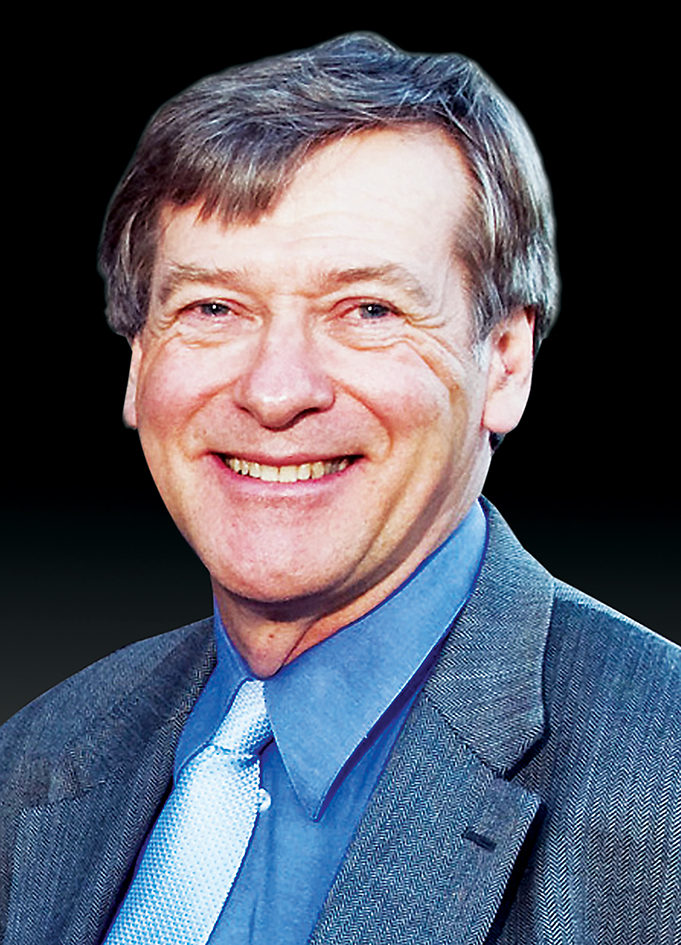
Roger D Blandford was born in 1949 in Grantham, Lincolnshire, United Kingdom and is currently Luke Blossom Professor in the School of Humanities and Sciences and Professor at SLAC National Accelerator Laboratory, Stanford University, USA. He obtained his Bachelor's degree in Theoretical Physics and his PhD from Cambridge University, UK in 1970 and 1974 respectively. He was a Charles Kingsley Bye-Fellow at Magdalene College (1972–1973) and Research Fellow at St John's College (1973–1976), Cambridge University. He then joined California Institute of Technology, USA, where he was successively Assistant Professor (1976–1979), Professor (1979–1989) and Richard Chace Tolman Professor of Theoretical Astrophysics (1989–2004). He was the Pehong and Adele Chen Director of the Kavli Institute for Particle Astrophysics and Cosmology (KIPAC) (2003–2013) and Professor of Particle Astrophysics and Cosmology (2003–2005) at Stanford University, USA. He was also a KIPAC Division Head, PPA Directorate at SLAC National Accelerator Laboratory (2005–2013). He is a member of the US National Academy of Sciences, the American Academy of Arts and Sciences and a Fellow of the Royal Society of London.
Roger D Blandford is one of the most outstanding all-round theoretical astrophysicists of his generation. He has made major contributions to an extremely broad spectrum of astrophysical problems, arguably placing him among the rare group of "universal" scientists. He has been one of the leaders in the modelling and interpretation of gravitational lensing. He has contributed to the interpretation of γ-ray data from the Fermi spacecraft and to the study of gravitational waves. His most important research contributions deal with the fundamental understanding of active galactic nuclei (AGN) and their relativistic jets.
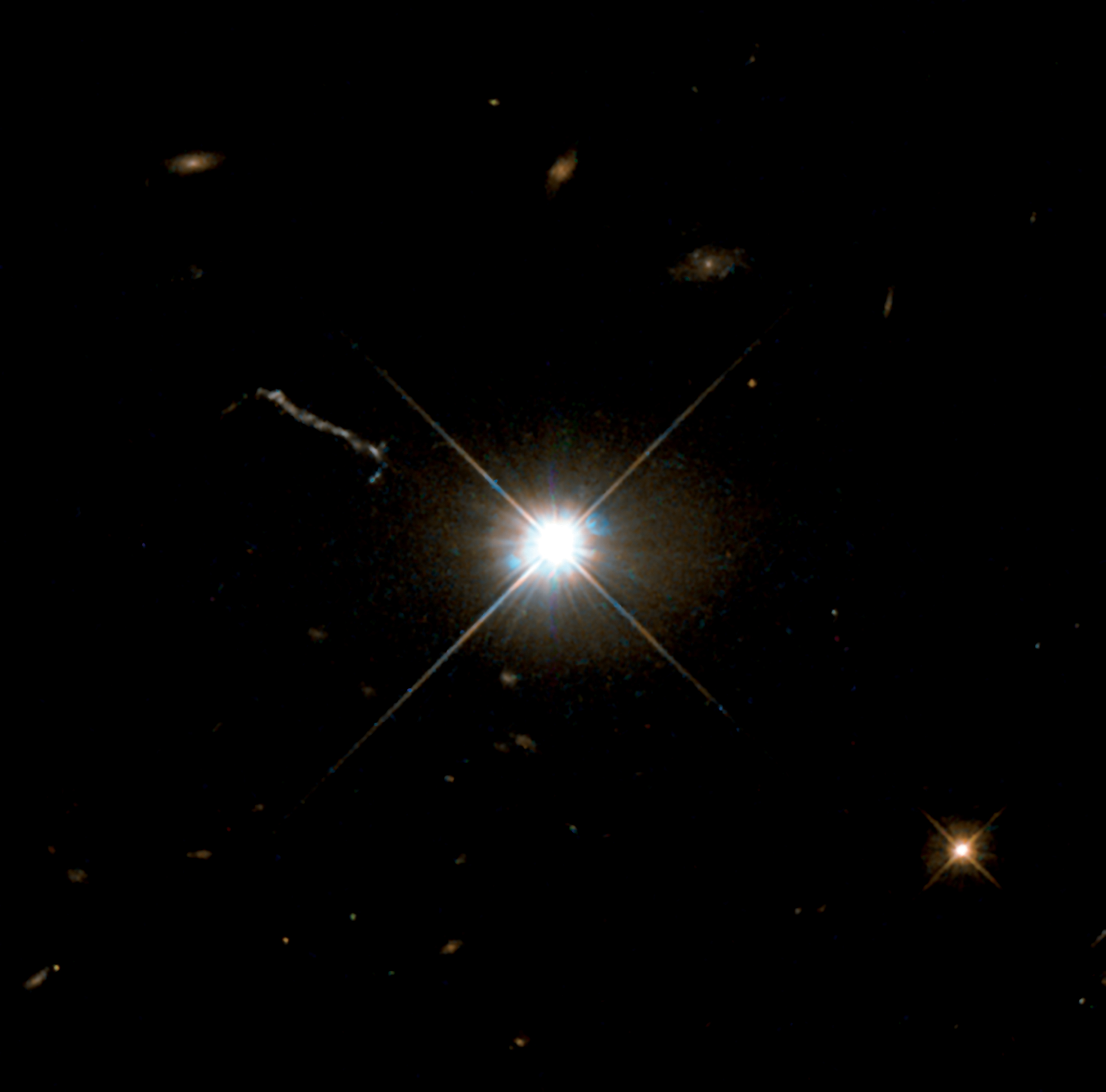
An active galactic nucleus (AGN) is a small central region of a galaxy that is much brighter than it would be in an average galaxy. A galaxy that holds one of these active nuclei is known as an "active galaxy". Quasars are the best-known representatives of AGNs.
The black hole, the accretion disk and the jets are important features of AGNs. The central black hole of an AGN has a mass from millions to billions solar masses. Material close to the black hole form an accretion disk. Matter spiraling into the hole heats up and emits energy over a broad range of the electromagnetic spectrum. Some of the disk material gets thrown out into jets, which are columns of charged particles beaming at high energies.
Captured by the Hubble Space Telescope, the image of quasar 3C273 (near the centre of the image above) showed a jet-shaped optical emission. 3C273 appears nearly point-like because its core outshone other parts of the galaxy. Four trillion times brighter than the Sun and at a staggering distance of 2.4 billion light-years in the constellation Virgo, 3C273 is one of the brightest quasars humans have ever observed.
The spectrum of a quasar 3C273 compared with that of a "normal" elliptical galaxy. The radiation from the elliptical galaxy mainly comes from the stellar population and is concentrated in a narrow section of the spectrum. Conversely, the enormous bandwidth of quasar spectra suggests that the radiation is non-stellar.
Jets are collimated beams of charged particles extending from compact objects such as black holes, neutron stars and pulsars. They are described as "relativistic" if the particles are accelerated to speeds close to the speed of light. Relativistic jets produce a signature type of radiation called synchrotron radiation.
Image Credit: NASA/JPL-Caltech/IPAC/Event Horizon Telescope Collaboration
As one of the most massive galaxies, M87 is an elliptical galaxy in the Virgo Cluster. A narrow jet of emission emerges from the glare of the galaxy's centre to nearly 5,000 light-years away at speeds larger than 99% of the speed of light. The centre of M87 is a black hole with 6.5 billion solar masses. The silhouette of the black hole was imaged by the Event Horizon Telescope in 2019.
Roger D Blandford and his collaborators originated key ideas leading to the spectacular multi-scale acceleration and collimation of relativistic jets, involving complex fluid-dynamical and electro-dynamical processes. In a process known as "magnetoluminescence" introduced by him and his collaborators in 2017, magnetic fields around an accretion disk are dragged and twisted by the spin of the black hole. Relativistic material is launched by the tightening of the field lines, producing high energy particles and γ-rays.
Image Credit: NASA, ESA, and A. Feild (STScI)
The accretion disc of hot plasma swirling around a supermassive black hole generates powerful magnetic fields which are twisted into a funnel shape. These field lines direct the outflow of high-speed plasma from the black hole's vicinity, forming a collimated jet.
One of Roger D Blandford most prescient contributions was the recognition that magnetic torques could extract energy from a spinning (Kerr) black hole — the Blandford-Znajek process.
In 1977, Roger D Blandford and Roman L Znajek proposed that the material accreting around a black hole would be magnetized and increasingly so as the material gets closer to the event horizon. If an electron remains outside the event horizon, the strong magnetic field will accelerate the electron to the point where it will radiate γ-rays. The black hole acts as a massive conductor spinning in the strong magnetic field produced by the accretion disk, where there is a voltage induced between the poles of the black hole and its equator. Energy is dissipated by the slowing down of the rotation of the black hole. For example, approximately 2.7 × 1038J of energy can be extracted from a 100 million solar mass black hole with a 1 Tesla magnetic field every second. It is larger than the global electricity consumption in a year.
A black hole is a compact celestial object such that in a certain region around it, objects even at the speed of light cannot escape. A rotating black hole is also called a Kerr black hole, which has two event horizons. Anything, including light, between the horizons can still escape from the black hole. However, once inside the inner event horizon, it can never escape.
Cosmic rays are the high-energy particles, primarily protons, that bombard the Earth's magnetosphere from space. Cosmic ray energy is usually measured in electron volts (eV) where 1 eV = 1.6 × 10-19J. The most energetic cosmic rays can have energies in the order of 1020eV, millions of times more energetic than the Large Hadron Collider's fastest particles. How can cosmic rays gain so much energy is one of the biggest mysteries in astrophysics.
Roger D Blandford and his collaborators proposed one of the most widely accepted mechanisms for explanation: diffusive shock acceleration. In this process, particles cross back and forward across the shock front as they scatter from magnetic inhomogeneities in the shock down and upstream regions. At each crossing, particles gain energy, speed up and finally are able to escape.
Image Credit: NASA's Goddard Space Flight Center
Driven by a magnetic field in supernova remnants, particles move across the shock wave. Particles gain energy with each round trip. After dozens to hundreds of crossings, the particle is moving near the speed of light and is finally able to escape, producing an energetic cosmic ray.
For the shock fronts of supernova remnants such as the Tycho Supernova, the mechanism can accelerate particles up to energies about 1014eV. The theory was proved observationally by detecting the γ-rays and synchrotron radiation emitted by accelerated particles. There is evidence that the shock fronts of large-scale galaxy clusters (e.g., Perseus cluster) are also responsible for faster particle accelerations.
The central continuum source of AGN heats and ionizes the gas nearby, producing emission line spectra. The strength of the emission lines is related to the strength of the continuum source. The radiation of the source varies, but it will take some time for the energy from the source to reach the gas cloud. The observed changes in the gas emission lines will be delayed compared to the continuum. Invented by Roger D Blandford and Christopher F McKee in 1982, the "reverberation technique" or "reverberation mapping" uses the temporal changes of line and continuum emission to explore the spatial structure of gas in the vicinity of distant supermassive black holes.
The technique is a now-standard technique used by many observers to study the central black hole in AGN. According to the AGN Black Hole Mass Database, black hole masses of more than 60 active galaxies up to billions of light-years away were measured by the technique over the last 35 years. The mass ranges from 0.1 million to several billion solar masses.
Image Credit: David W. Hogg, Michael R. Blanton, and the Sloan Digital Sky Survey Collaboration
NGC 4151 is one of the galaxies studied by astronomers using the "reverberation technique". When the X-ray source near NGC 4151's black hole flares up, the accretion disk echoes the emission later. By timing the delay, it is estimated that the size of the disk is comparable to that of our Solar System and the black hole mass is around 50 million solar masses.